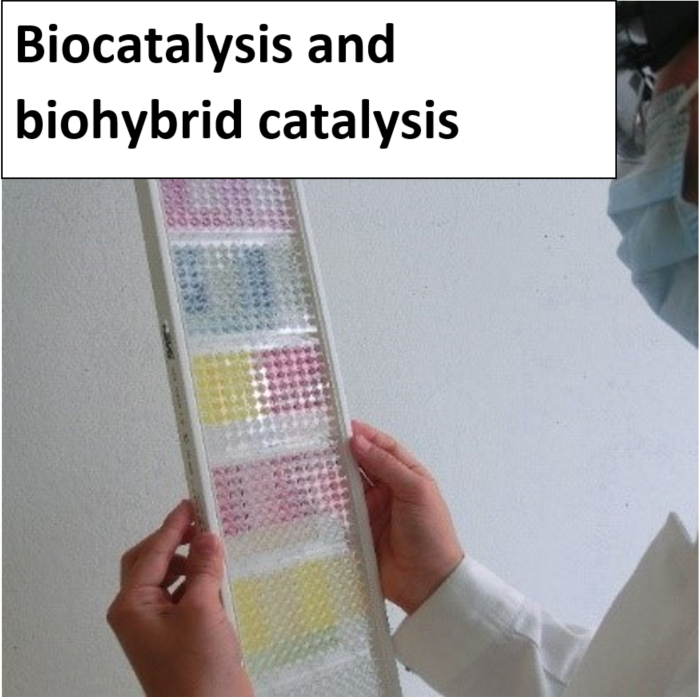
To replace a fossil based industry, environmentally friendly, efficient and economical processes based on new synthesis methods and strategies are needed. We are dedicated to this field of research, focusing on enzymatic synthesis methods as well as the combination with chemical or electrochemical methods, called biohybrid catalysis.
Nature provides a variety of biocatalysts (enzymes), all of which have the intrinsic ability to convert biogenic substrates. However, approaches for the conversion of biomass into basic and fine chemicals require biochemical parameters that differ significantly from those in the enzymes’ natural habitats. The enzymes must be compatible with each other, but also tolerate all intermediates that occur, high product concentrations and solvents.
In order to optimise them for this and for the corresponding biotransformations, we have to tailor many enzyme properties, such as (thermo)stability, specific activity, substrate specificity, cofactor specificity and inhibition. The focus is always on an application, but we also try to understand the molecular understanding of the catalysis processes in the enzyme through enzyme engineering. Successful examples from our work include stabilising enzymes to function at high temperatures, optimising general activity, changing cofactor specificity and using non-natural cofactors.
Crucial to the success of directed evolution is the quality of the libraries and the efficiency and accuracy of the screening procedures. We develop new mutagenesis and recombination strategies as well as chemical, enzymatic or physical high-throughput assays and selection methods. The assays are established in the classical 96-well format (medium to high throughput) on our robotic platform as well as for ultrahigh throughput applications on our microfluidic systems.
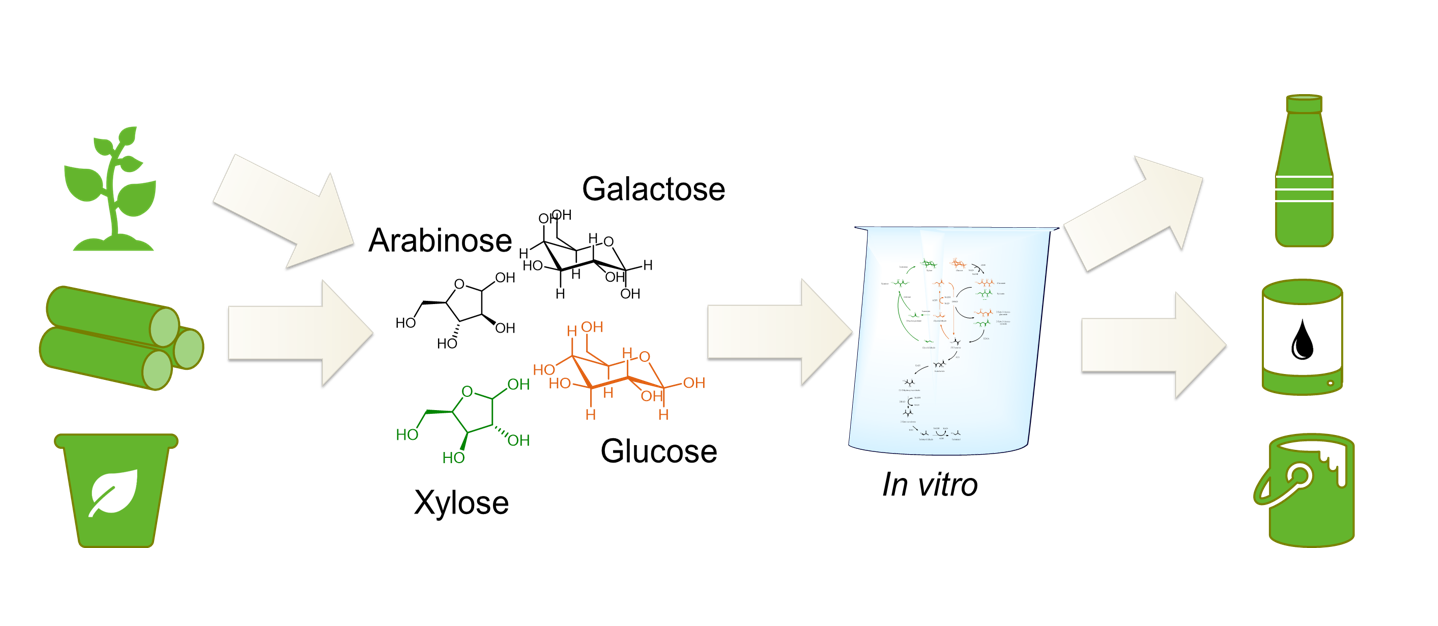
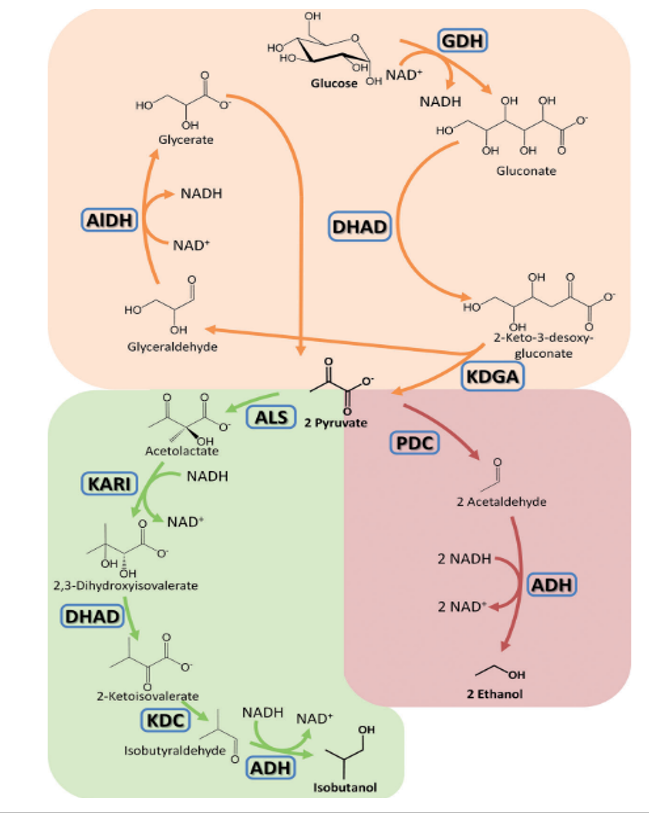
Multi-enzyme catalysis means using several enzymes at the same time without having to isolate the resulting intermediates before they are used for the next reaction. This is particularly possible with enzymes, in contrast to chemical catalysts, because they can work effectively under similar conditions or can be modified to be compatible with each other. Our goal is to use multi-enzyme catalysis to produce platform and fine chemicals from sugars or other molecules that can be obtained from renewable resources.
For the development and optimisation of multi-enzyme catalysis, we are investigating
- The intrinsic properties of the enzymes involved (activity, specificity, stability) and optimise them if necessary (see enzyme engineering).
- Analytical methods such as HPLC, GC, MS, NMR or enzymatic assays, for the detection and quantification of the intermediates, products and, if necessary, by-products, in order to understand the interplay of the enzymes in multi-enzyme catalysis and to identify their weaknesses and strengths.
- Mathematical models (in cooperation) to make predictions about bottlenecks and optimal conditions for multi-enzyme catalysis according to the research question.
Various multi-enzyme cascades have already been established at the Chair and are being continuously developed:
In an artificial enzyme cascade developed by us, pyruvate is produced from glucose with four enzymes and the classical glycolysis, which requires 10 enzymes for this, is greatly shortened and simplified. Pyruvate, in turn, can be produced as a starting molecule for a number of interesting products, each with its own cascades (amino acid, isobutanol, etc.).
Other starting molecules for our multi-enzyme catalyses are xylose, which can be obtained from hemicellulose, or glycerol.
In the context of multi-enzyme catalysis, we also deal with the coupling of enzymatic with chemical or eletrochemical reactions, the design of new more stable or cheaper cofactors and, necessarily, enzyme engineering.
The new field of biohybrid enzyme catalysis aims to combine catalytic methods from different fields with enzyme catalysis. Our main focus is on chemo-enzymatic synthesis and biohybrid electrocatalysis for the generation of basic and fine chemicals from renewable raw materials.
There are already a number of examples where such biohybrid approaches are being explored. A wealth of organic and inorganic chemical catalysts and electrochemically used electrode materials exist. Nevertheless, they generally lack compatibility with enzymes and biogenic substrates and their associated solvents. On this basis, we are researching chemo-enzymatic and electro-enzymatic processes for the efficient and targeted conversion of complex biogenic raw materials. We have had initial success in combining chemical oxidation by molecular oxygen with biocatalytic dehydration reactions. As an example, four different 2-keto-3-deoxy sugar acids could be obtained by combining a gold catalyst retained in a continuous reactor with an immobilised dehydratase. Furthermore, the continuous combination of electrochemical reactions with bioenzymatic product generation is being researched in depth by means of process analyses and electrode development.
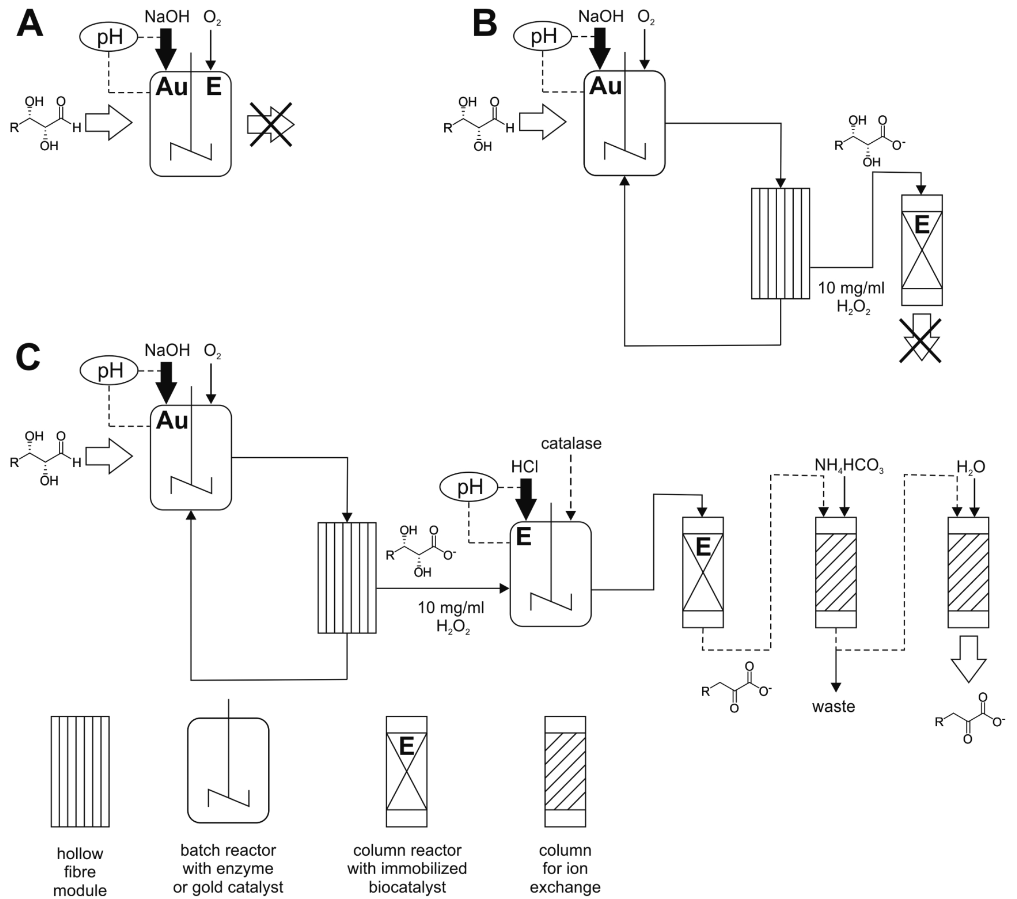
Metabolic engineering refers to a variety of different methods to optimise the production of valuable target molecules from cheap and simple substrates using microbial production organisms by altering or rearranging the genetic information of the microorganisms. This mainly involves improving metabolic fluxes by, for example, preventing the production of unwanted by-products or optimising critical bottleneck steps along an enzymatic cascade. In addition, we are also establishing new, artificial production pathways in our microbial factories in order to be able to produce completely new products, such as fine chemicals, by fermentation.
Our research is not only focused on optimising conventional laboratory organisms, but also alternative production platforms such as Paenibacillus polymyxa, which can produce interesting products. We use highly efficient, modern tools such as CRISPR/Cas9 to target the genome of these organisms and improve production. In addition, we are also developing new tools to regulate complex metabolic cascades.
Using green hydrogen as a cheap clean energy source as an alternative to carbon-based fuels reduces carbon dioxide emissions and contributes to reducing global warming. Hydrogenases are attractive biocatalysts for the production and utilization of hydrogen. As a part of the TUM international Future Lab (REDEFINE H2), we aim to produce green hydrogen from biogenic materials like sugars using engineered hydrogenases. Furthermore, we work on developing superior hydrogenases variants that can be applied in industrial processes, where hydrogen can be employed to drive the synthesis of biofuels.
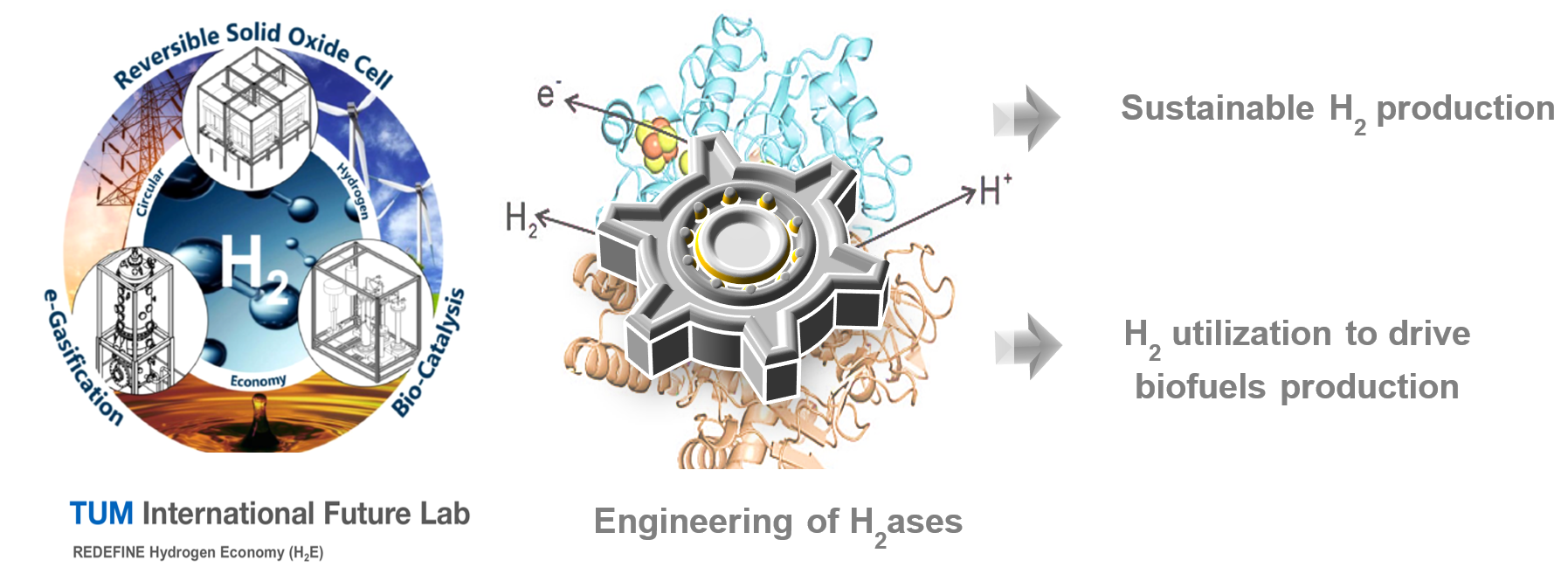